Product: Thermal neutron generator
Model: DD109M.1 and DD109M.5
Generator type | Type and model number(s) | Specifications |
---|---|---|
![]() |
Thermal, internal
target, actively pumped
|
|
Summary of this thermal neutron generator
This generator is capable of 5x109 n/s. It is very similar to the DD110M.2. The difference is that it is configured to be used with a smaller High Voltage Power Supply. The device is especially well suited to sample activation for Delayed Gamma Neutron Activation Analysis (DGNAA) and has also been used by several groups for thermal neutron imaging.
Overview of Adephi's thermal neutron generators
All of Adelphi’s neutron generators operate using a microwave driven Electron Cyclotron Resonance (ECR) ion source. Ions produced in the ion source are accelerated towards a target. The ions implant into the target. Subsequent ions can collide with ions that have been embedded in the target. When accelerated by a sufficiently high voltage, these ions can undergo nuclear fusion, leading to several nuclear reactions. The two relevant reactions for Adelphi’s neutron generators are:
- D+D=He-3 + n (2.45 MeV)
- D+T=He-4 + n (14.1 MeV)
D denotes deuterium, T denotes Tritium, “He” indicates a helium nucleus either the isotopes He-3 or He-4, ndenotes a neutron and the energy of the neutron is stated in MeV. Generators that contain tritium produce neutrons with a higher energy than those that only contain deuterium gas. This leads to different penetrating power, imagingcontrast,and different activation of elements due to the difference in collision cross sections of elements (isotopes) as a function of energy.
The ions are produced in the ion chamber when the microwave source is energized. The RF source can be either pulsed or operated Continuous Wave (CW). Minimum pulse lengths of 50 microseconds have been achieved.
Adelphi’s D-D neutron generators contain deuterium gas. We offer “D-D sealed” and “D-D open” variants. The D-D open systems can be high yield (up to 10^10 n/s), the “D-D sealed” generator is lower yield and as the target on the exterior surface of the generator. Customized generators that are open systems with the target on the exterior surface have been built.
Adelphi’s thermal neutron generators (the DD109-M and DD110-M) produce thermal or epithermal neutrons. Within the generator, the D-D reaction is used to produce 2.45 MeV neutrons. These neutrons are then thermalized with a High Density Polyethylene (HDPE) moderator within the neutron generator. This leads to a relatively large “spot” of thermal neutron emission on the surface of the generator.
The flux of neutrons has been measured using gold foil activation as a function of position across the generator.
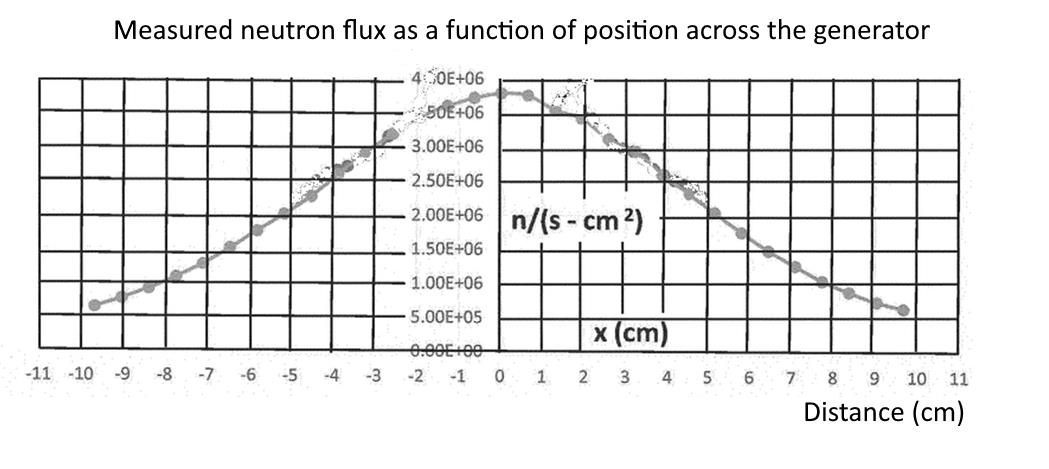
This flux of around 4x106 n/s/cm2 is high for a laboratory neutron source. With proper shaping of moderators using appropriate Beam Shaping Apparatus (BSA), it is possible to more than double this flux as described in Adelphi’s US Patent US11090509. The neutron flux that this generator produces is essentially the same as the neutron flux in the world’s first nuclear reactor, the Chicago Pile 1 (CP-1), which is estimated to have a flux of 106 to 107 n/cm2/s. The CP-1 had this flux over a much larger volume of course. Today’s reactors have much higher fluxes of 1013 to 1015 n/cm2/sec.
The DD110 generators, despite being very high flux for their size, and finding a wide range of applications, are not a replacement for all nuclear reactor applications. This neutron flux is, however, comparable to fluxes available at reactor beamlines. At these facilities, an opening or port lets neutrons out of the reactor. These neutrons then travel along pipes where they can be used for research. The flux is lower at the station on the beamline than in the reactors core, due to the distance from the reactor (and the inverse square law). So, for some applications Adelphi’s thermal neutron generator sources offer an alternative to reactors.
This class of generators uses a bottle of deuterium gas and continuously pumps the system using a turbo-molecular (‘turbo’) pump to maintain the vacuum. Gas flows from the bottle through the ion source and is exhausted out of the vacuum pump. The flow rates are very low, typically 1 to 5 standard cubic centimeters per minute (SCCM), so there is minimal volume of deuterium exhausted from the vacuum system’s roughing pump. This approach of actively pumping on the vacuum system, allows vacuum in the acceleration region to be maintained at a much lower pressure than the ion source – enabling the mean-free-path in the acceleration region to be long compared to the ion-source to generator-target distance. This allows this class of generators to achieve near-theoretical neutron yield efficiencies (which we express as neutrons produced per Coulomb of deuterium ions). The ECR ion sources used in these generators produce mostly atomic species of ions (as opposed to molecular species) which is an important factor in enabling these generators to produce neutrons so efficiently. The ion sources are powered by 2.45 MHz Radio Frequency (RF) radiation (otherwise known as microwaves). Adelphi typically uses solid state microwave sources, although magnetrons can also be used.
These generators are very serviceable, and any failing components can be replaced. This essentially provides the generator with an unlimited lifetime. Maintenance is important when high yield generators are run at full output for long durations. Some of our customers operate these generators 24-7 for several months in between scheduled maintenance (replacing gas bottles and checking on cooling levels).
The generators need a supply of deuterium gas to operate the ion sources. The low-yield generators operating at 10^8 n/s require a gas flow rate of around 1 Standard Cubic Centimeters per minute (SCCM) which is metered into the generator using a Mass Flow Controller (MFC). A small gas ‘lecture’ bottle provides around 800 hours of operation. A high-yield generator operating at 1010 n/s has a higher flow rate of around 5 SCCM so a small lecture bottle would last around 160 hours, therefore, a larger bottle is typically employed to reduce the duration between bottle replacement.
The generators also have a closed-loop cooling system. The size of the cooling system is directly related to the neutron yield. This cooling system is provided with the generator and transfers the heat energy into the room requiring your building’s HVAC (Heating Ventilation and Air Conditioning) system to be able to accommodate the heat load. For a 108 n/s D-D based generator, this is less than 1kW, for a 2x1010 n/s D-D generator, this can be closer to 10kW. Depending on the generator variant, the coolant may be distilled water, or it could be an insulating liquid such as de-ionized water or a fluorinated liquid such as fluorinert FC-3283.
Images
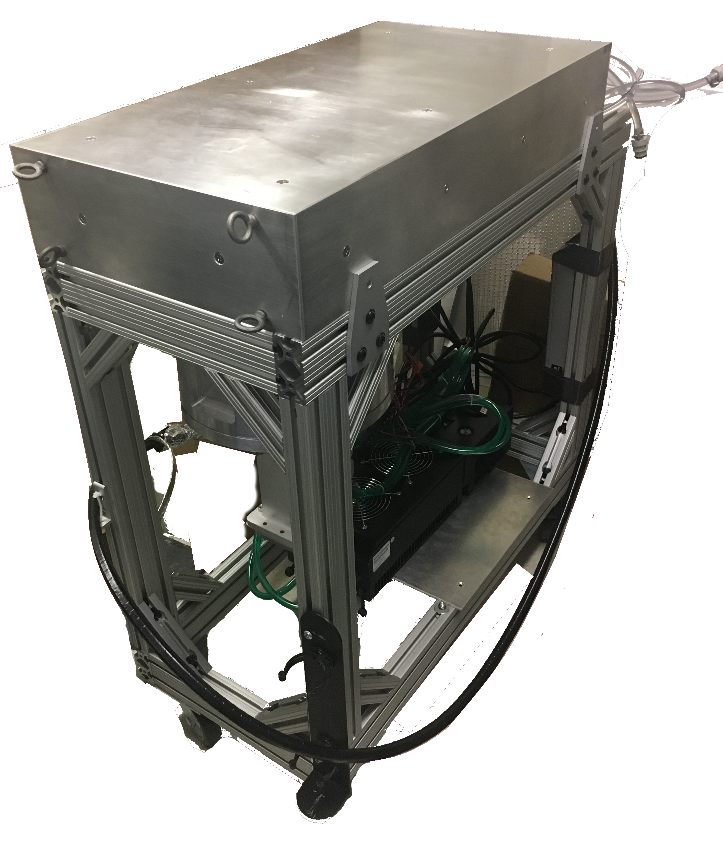
DD109M.5 Thermal
Thermal neutron generator. Mid 10^9 n/s yield
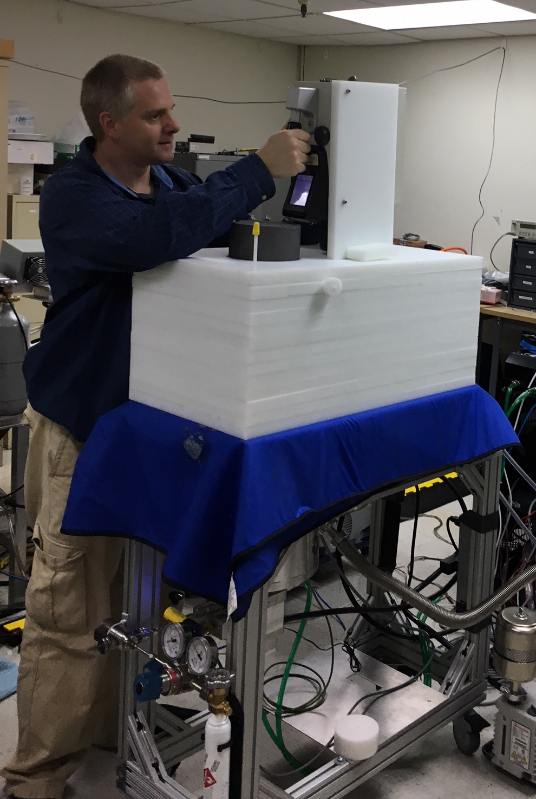
DD109M.5 Thermal
Thermal neutron generator. Mid 10^9 n/s yield
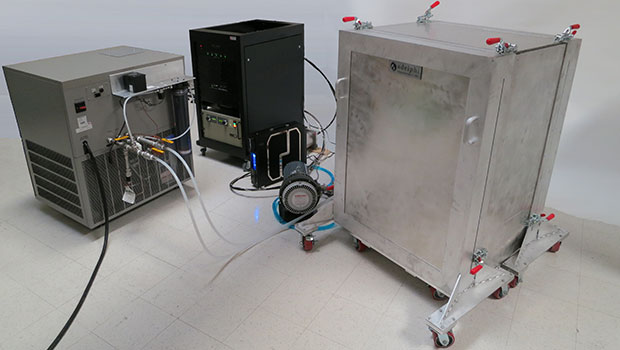
DD109M.5 Thermal
Thermal neutron generator. Mid 10^9 n/s yield
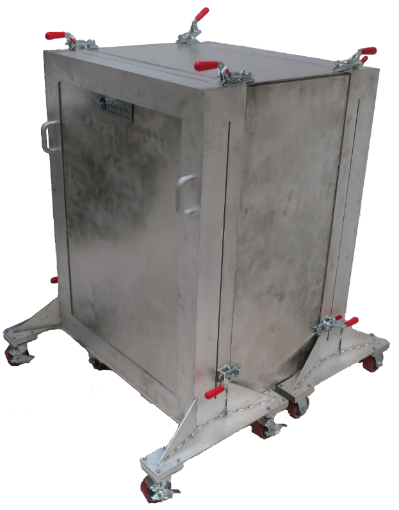
DD109M.5 Thermal
Thermal neutron generator. Mid 10^9 n/s yield
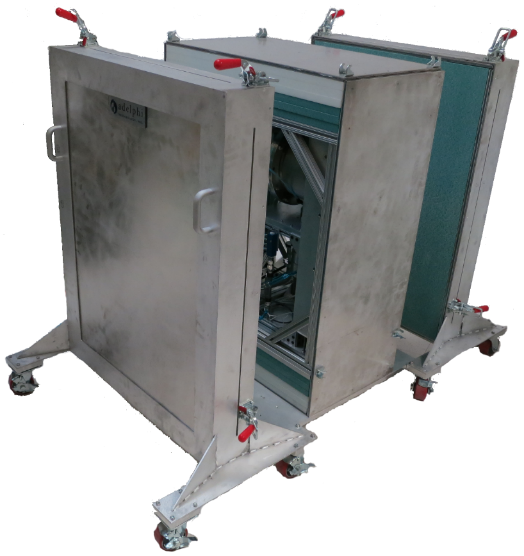
DD109M.5 Thermal
Thermal neutron generator. Mid 10^9 n/s yield
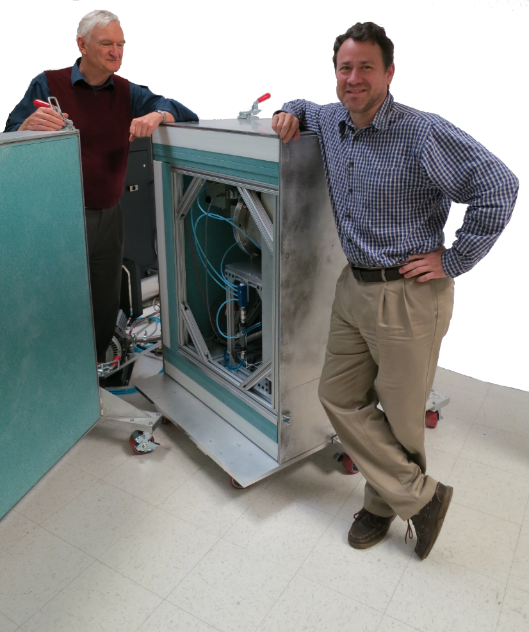
DD109M.5 Thermal
Thermal neutron generator. Mid 10^9 n/s yield